Chapter 10
Applications in Biology
Note to Lay Readers
This is the second of two important, but difficult, chapters. If you start to bog down, you may freely skip ahead to the next chapter.
Protein to Protein Communication
The proteins of a cell perform all the functions needed for a cell’s survival just as people do for a city. A city with millions of inhabitants would descend into chaos if communication were not possible between its inhabitants. So should we expect the same to happen to a cell if its millions of proteins could not communicate with each other.
So how do the proteins within the cell communicate with one another?
In the mind signature, there is a double bond between the oxygen atom and the carbon atom, and this double bond makes the oxygen atom chemically stable. For this reason, the oxygen atom of a mind signature will not form covalent bonds with outside atoms. However, these oxygen atoms can and do attach themselves to other proteins via hydrogen bonds.
Hydrogen bonds are simple electrostatic attractions, they are not covalent bonds. For this reason, minds cannot govern across hydrogen bonds. Nevertheless, hydrogen bonds still have a lot going for them as communication pathways.
Figure 19 shows four hydrogen bonds (vertical dashed lines) connecting two proteins, where one protein extends across the top of the figure and the other protein extends across the bottom. In the figure, the individual minderelles of the proteins are enclosed by blue lines. Notice how the minderelles overlap each other at the shared bonds.
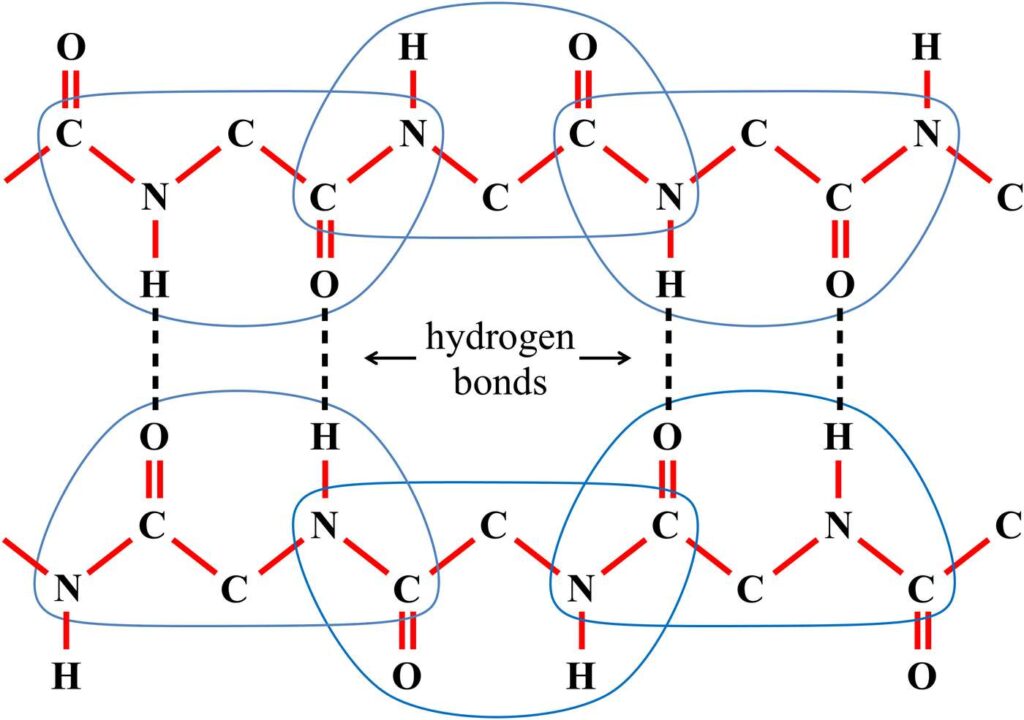
Figure 19. Two proteins are connected together by the four hydrogen bonds shown above as vertical dashed lines.
Notice that in each hydrogen bond, the hydrogen atom is positioned between a nitrogen atom and an oxygen atom, both of which are much more massive than the hydrogen atom. Due to this significant mass difference, the vibrational frequency of the hydrogen atom is much higher than the vibrational frequency of either the nitrogen atom or the oxygen atom.
Notice also that this rapidly vibrating hydrogen atom belongs to a minderelle. The mind on the nitrogen side can impose a length lock on the N–H bond. This length lock will drop the vibrational frequency of the hydrogen atom suddenly and dramatically to that of the nitrogen atom. This means that the N–H bond can now be locked and unlocked to generate easily detectable variations in the vibrational frequency of the hydrogen atom.
Because the vibrating hydrogen atom is electrostatically attached to the oxygen atom, the hydrogen vibrations will create a varying external force on the oxygen atom. Because minds are informed of their surroundings by external forces acting on their minderelles, the mind governing the oxygen side of the hydrogen bond will detect the dramatic changes in the hydrogen atom’s vibrational frequency.
The dramatic changes in the vibrational frequency of the hydrogen atom due to N–H length locks can now be used to generate the 1’s and 0’s needed to make communication possible between the proteins.
Let us take a moment to review the three factors that make the hydrogen bond such a good communication link: (1) The hydrogen atom is not attached to any other atoms that would introduce noise or bleed off signal. (2) The nitrogen, hydrogen, and oxygen atoms generally lie along a straight line, maximizing the hydrogen atom’s vibrational effects on the oxygen atom. (3) The change in the vibrational frequency of the hydrogen atom caused by locking and unlocking the N–H bond is dramatic, so these frequency changes are easily detected.
Each hydrogen bond only provides a one-way communication pathway because the vibrating frequency of the hydrogen atom can only be controlled by the nitrogen side of the hydrogen bond. However, because hydrogen bonds are paired together in opposite directions, as shown in Figure 19, each hydrogen bond pair will give us a full bi-directional communication link between the two minderelles. For this reason, we will count a parallel opposed hydrogen bond pair as a single, bi-directional communication link in our later discussions.
It is worth noting that if the hydrogen atom of a hydrogen bond were replaced with the larger deuterium atom, the higher mass of the deuterium would reduce the difference between the locked and unlocked vibrational frequencies, and would thus reduce the data transmission rate of the hydrogen bond communication pathway.
Governing the φ and ψ Dihedral Angles
The three Greek letters φ, ψ, and ω are used to identify the molecular bonds along the backbone of a protein, as shown in Figure 20. Recall that a dihedral angle refers to the angle formed by three consecutive bonds, and each dihedral angle is identified by the name of the middle bond (this was illustrated in Figure 9).
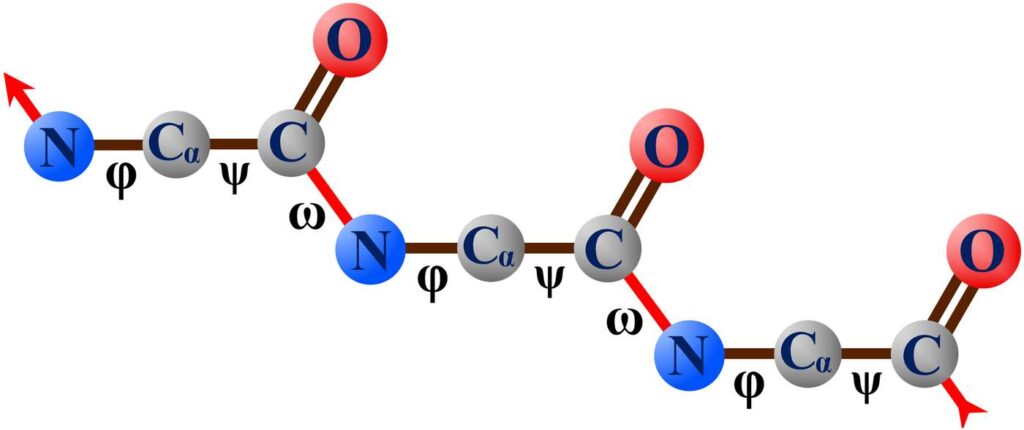
Figure 20. Scientists use the Greek letters φ, ψ, and ω to identify the bonds and dihedral angles of a protein backbone. (In three dimensions, the φ and ψ bonds do not lie in a straight line.)
For proteins to fold and maintain their shape, the dihedral angles of the protein backbone must not be free to rotate uncontrollably when the protein is acted upon by external forces. This is not a problem for the ω dihedral angle because the ω dihedral angle remains fixed due to the characteristics of the ω bond itself.
However, both the φ and ψ dihedral angles can vary across a wide angular range that is free of any restoring force. This means that for the protein to be functional, the minderelles making up the protein will have to have governing control over the φ and ψ dihedral angles. Recall from Chapter 5 that a mind can only lock a dihedral angle if the mind has governing control over the three bonds that form the dihedral angle.
If we examine the protein backbone in Figure 20, we can see that each mind will have full governing control over the three bonds that make up the φ and ψ dihedral angles due to our earlier finding that minds govern their peripheral bonds. (The ω bonds are the connecting bonds between the amino acids.) Therefore, the finding that minds govern their peripheral bonds has also given each minderelle full governing control over its own dihedral angles, so working together, the minderelles will be able to fully control their protein’s shape.
Protein Folding Using Geometry Locks
After a protein is formed, it spends time in a folding area where the protein folds itself into its functional shape, sometimes with the help of a chaperone protein. Many types of forces are used to advance the folding process, including the microcurrents within the folding area, the hydrophobic and hydrophilic interactions of the R-groups, Van der Waals forces, and the mind’s own geometry locking forces.
Out of all these forces, the geometry locking forces are singularly the most important. The protein primary will lock and release the protein’s dihedral angles in a way that takes advantage of the external forces at each moment in time to advance the folding process. We will now cover protein folding in a little more detail.
After the protein’s formation, the protein primary will lock all the dihedral angles of the protein. The primary will then release the locks on its dihedral angles in a predetermined order so that the folding process will follow the correct folding sequence. However, each dihedral angle will only be unlocked when the external forces acting on the protein have a direction and magnitude that will correctly move the folding process forward.
To promote the folding process, cells will keep the conditions in the protein folding area within acceptable limits. As an example, let us consider the forces created by the random microcurrents in the folding area. If these microcurrents are produced by temperature gradients, then all the factors that affect the temperature gradient in the folding area will be important for the cell to control.
The factors that influence the temperature gradient will include the distance between the heat sources and the heat drains, as well as the conditions that could affect either the supply of heat or its removal on the cold side. If heat is removed by conduction through the cell walls and then carried away by outside blood flow, then changes in the volume or speed of the adjacent blood flow would be critical factors. Changes in the insulating characteristics of the cell wall, or other similar factors, could also bring about serious consequences.
Aside from any physical assistance that might be offered by chaperone proteins, they may also serve as local heat generators to maximize microcurrents, or they may create other highly localized gradients immediately adjacent to the newly formed protein.
Nevertheless, even if acceptable conditions are maintained, there will always be some probability that any given protein will not be provided with the folding forces needed to fold properly within the allotted time. The ratio of proteins that fail to fold properly to those that do fold properly gives us a folding failure rate. The higher the folding failure rate, the higher the probability that misfolded proteins will encroach into the folding area and interfere with other folding proteins, thus creating a risk of folding failure runaway.
However, as long as the cell has maintained proper control over all the factors that affect the folding area, the geometry locking capabilities of the primary will typically be sufficient to successfully direct the folding process.
The Minderelle’s Communication Links
We have now found that each minderelle in a protein can form up to three bi-directional communication links. Two of these links pass through the shared ω bonds that connect adjacent neighbors, while the third communication link passes through the hydrogen bond pairs that connect proteins together. Figure 21 shows these three communication links. The arrows in the figure indicate the direction of information flow for each of these connections.
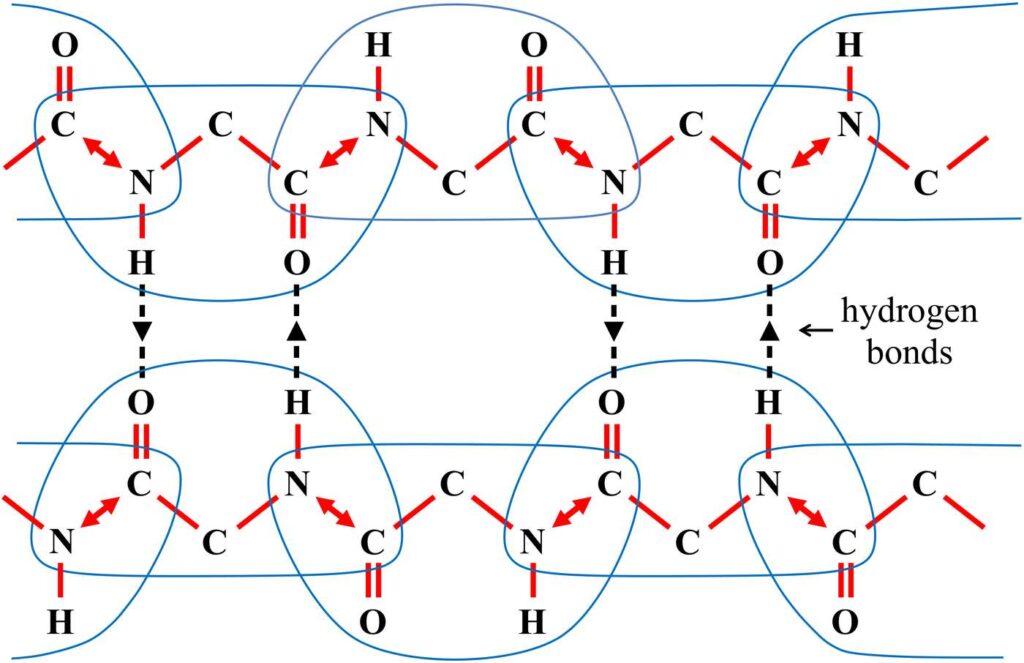
Figure 21. The two proteins here are connected by hydrogen bonds. The minderelles are outlined with blue lines. The arrows indicate the direction of information flow through the three communication links.
Minderelles will not be able to use planar angle locks or dihedral angle locks for communication purposes because there would be no reliable method for one minderelle to determine whether the angles of a neighboring minderelle are locked or unlocked. This means that all communication between minderelles will have to be carried out with length locks. Because length locks have only two possible states – locked and unlocked – all communication between minderelles will take place using binary 1’s and 0’s in the same way that today’s computers and electronics move and process information using 1’s and 0’s.
We have now found that, in general, minderelles communicate using length locks while planar and dihedral angle locks are dedicated to functional purposes. That is, communication between minderelles takes place independently from the functional tasks being carried out.
In Chapter 5, we found that minds receive information about their surroundings through outside forces acting on their minderelles. We are now finding that the most important outside forces acting on a minderelle are the information bearing forces from neighboring minderelles.
Beta Sheets
The previous figure showed two proteins joined together by hydrogen bonds. In cells, these hydrogen bond connections between different proteins are common and often connect multiple proteins together, as shown in Figure 22. These two-dimensional arrangements are called beta sheets. Notice the many hydrogen bonds throughout the beta sheet, and recall that proteins communicate with one another across these hydrogen bonds. Thus, beta sheets in cells are hubs that enable communication between multiple proteins. We will later find that beta sheets are also used to improve communication within single, complex proteins.
In Chapter 12, we will find that a beta sheet is used to connect the human primary to the human brain. That is, we will find that a beta sheet communication network makes it possible for our single minderelle to receive all the sensory data from our brain, and to communicate command decisions back out to the brain.
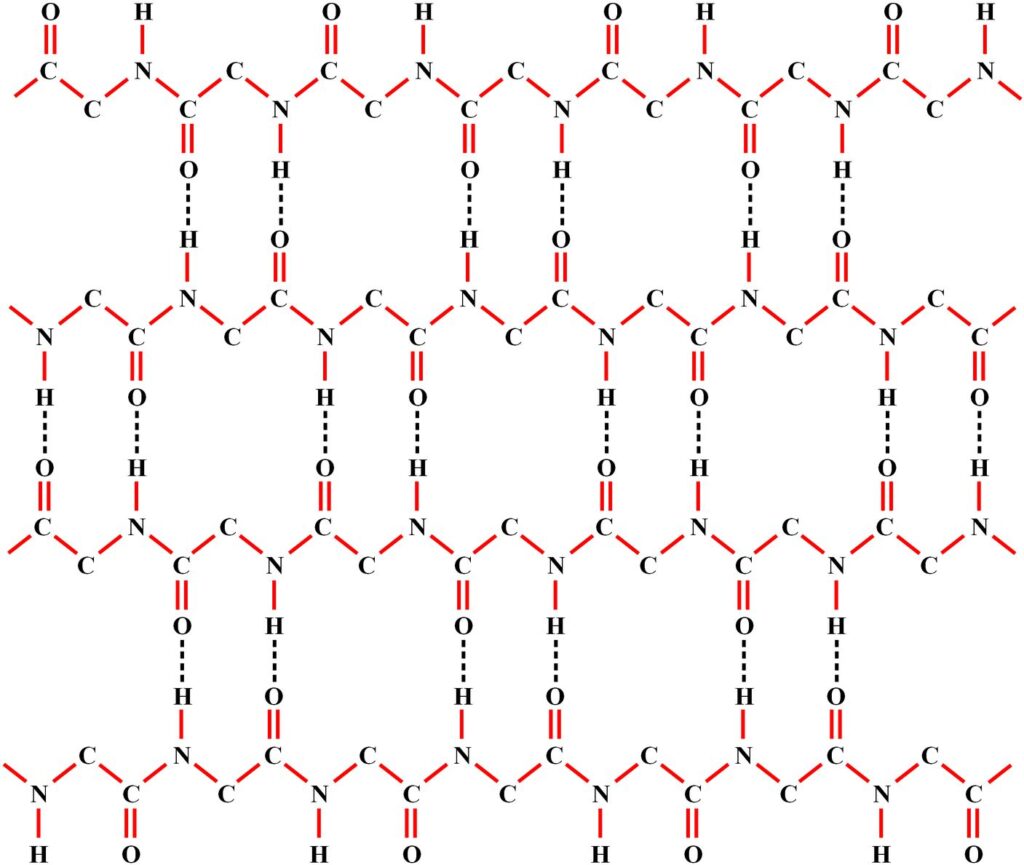
Figure 22. The amino acids here form a beta sheet, a common structure found in cells.
Amino Acids as Neurotransmitters
Neurotransmitters are substances in the brain that transmit signals from one neuron to the next across synapses. Glutamate and other amino acids are commonly used by neurons as neurotransmitters. Because these neurotransmitters are amino acids, according to this free will paradigm, they are also fully conscious, thinking, intelligent individuals. This means that these neurotransmitters are likely acting as intelligent messengers rather than as simple signal transmitters. We will expand on this understanding in Chapter 13.
The Cytoskeleton
We stated earlier that at least some cells have primaries. This would mean a single minderelle within the cell is making the decisions on the actions to be taken by the cell. For these cells to be functional, there would have to be a communication network that brings information from the cell’s surroundings to the primary, and that also carries commands from the primary back out to those areas of the cell that are responsible for putting the cell into motion.
Thus, the cytoskeleton may be more than a transportation, distribution, and support structure. The cytoskeleton may also function as a communication network. We would expect the cell primary to be at a focal point within the cytoskeleton, and we would further expect this point to be close to the center of the cell where it would be the most protected. We will discuss what makes one specific minderelle the primary of a humanon in Chapter 12.